By Arabel Yap, Dip VeT
INTRODUCTION
Diagnostic imaging has consistently played a crucial role in veterinary medicine since the late 1900s (Meomartino et al., 2021) with the ability to greatly enhance the efficiency of clinician diagnosis. Despite radiography and ultrasonography asserting their position as the more fundamental options, given their accessibility and economic cost-effectiveness, there is a growing trend worldwide in the increased utilisation of advanced diagnostic imaging such as Computed Tomography (CT) and Magnetic Resonance Imaging (MRI) in veterinary practice. This shift can be attributed to these two modalities’ enhanced, non-invasive, diagnostic capabilities.
Computed Tomography (CT) proves extremely advantageous by delivering a wealth of information with the only trade-off being a minimal duration of general anaesthetic. With conventional radiology, we often face the hindrance of obstructive overlying organs or tissues that superimpose internal structures. There is an element of uncertainty as the images produced only provide a two-dimensional picture and may occasionally be insufficient in achieving a diagnostic conclusion due to lack of visibility.
CT imaging attains superior bone definition and anatomical location. It provides detailed cross-sectional images of the body, allowing veterinarians to visualise internal structures more accurately and even generate 3D images with the help of reconstruction algorithms. Some of the common applications of veterinary CT include:
- Musculoskeletal and joint disorders
- Angular Limb Deformities (ALD)
- Canine Elbow Dysplasia
- Soft tissue abnormalities and neoplasia
- Shunts and Vascular Anomalies
- Nasal/Oral lesions
MUSCULOSKELETAL AND JOINT DISORDERS
Angular Limb Deformities (ALD)
CT is increasingly used to diagnose and assess bone angulation in patients with ALD. Axial or helical acquisition can generate three-dimensional models with volume rendering or multiplanar reconstruction (MPR) of the affected bones to improve visualisation and orientation for surgical planning. It can also help quantify the degree of angular deformity in the limbs and assist in preoperative preparation.
Canine tibial or radial torsion with valgus or varus deformities requires the measurement of angles between multiple planes. CT has been demonstrated to be more accurate than traditional radiography where two-dimensional angular measurements may vary as it depends significantly on the standardisation of positioning and alignment of the X-ray beam (Brühschwein et al., 2023).
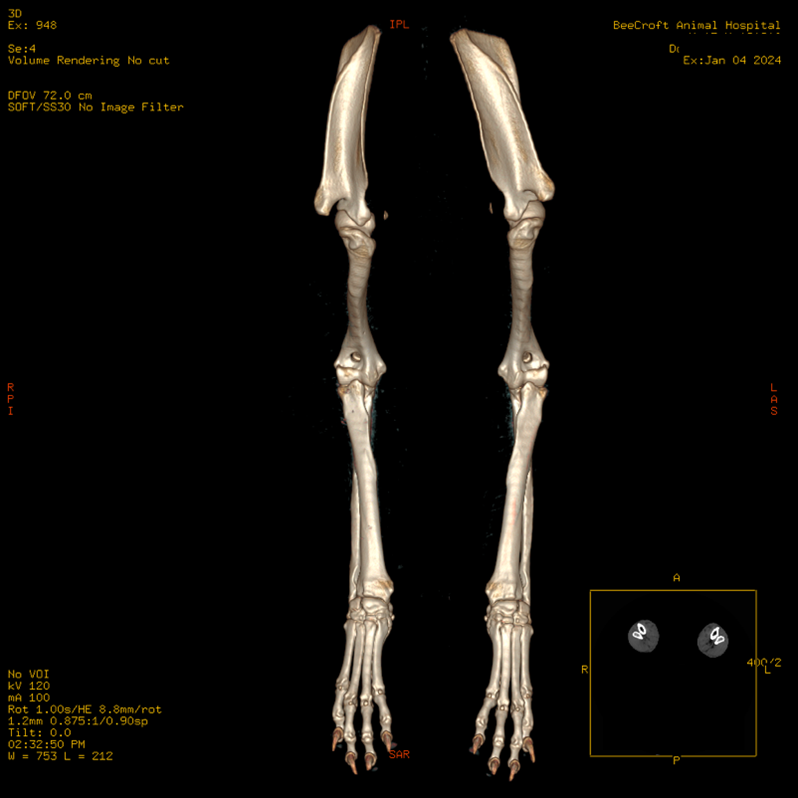
Figure 1. Dorsal plane volume rendering of the whole antebrachium with both the full elbows and carpi.
Canine Elbow Dysplasia (CED)
Canine elbow dysplasia is one of the more common skeletal disorders. Since CED is hereditary, the sensitivity of CT imaging can provide detection in dogs as young as 14 weeks old (Lau et al., 2013). Although ununited anconeal processes and osteochondrosis can be successfully diagnosed on radiographs, multiplanar reconstructions offer sagittal and dorsal planes that are essential in accurately identifying certain pathological conditions associated with CED including joint incongruity and medial coronoid process fragmentation (Hebel et al., 2021).
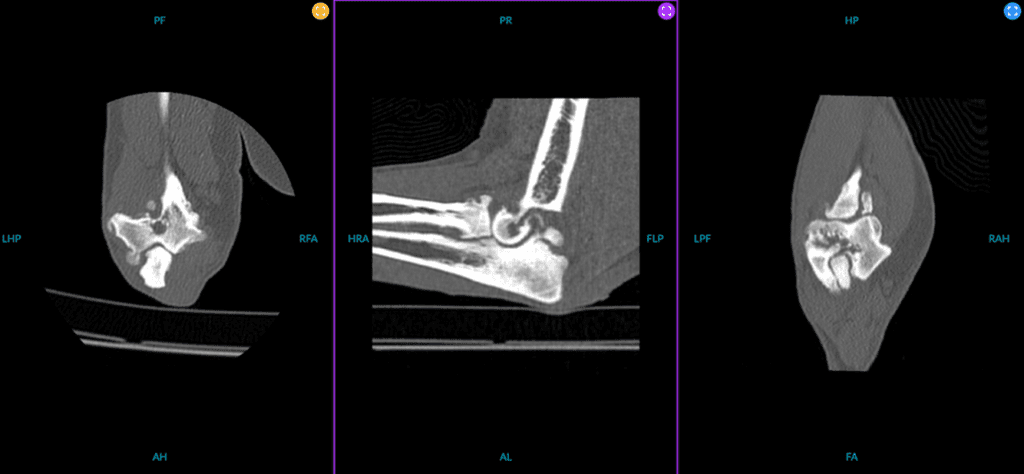
Figure 2. Multiplanar reconstruction of a patient with elbow dysplasia characterised by medial coronoid fragmentation.
SOFT TISSUE ABNORMALITIES AND NEOPLASIA
Common clinical indications for CT scans encompass tasks such as tumour staging, delineation of surgical margins, and evaluation of metastatic disease, often employing CT angiography (CTA). Initial diagnostic steps typically involve ultrasound due to its accessibility and cost-effectiveness. However, the inherent drawback of ultrasound lies in its relatively restricted field of view, limiting the simultaneous assessment of larger areas or multiple structures. This limitation poses a challenge in accurately pinpointing the origin of mass lesions, often prompting subsequent CTA scans to assist in precisely determining anatomical locations and vascular associations crucial for surgical planning.
Positive iodinated contrast has a high atomic number, effectively absorbing X-ray beams. The increased blood flow in tumours often results in greater contrast agent accumulation, making tumours more conspicuous in the images. When injected into the bloodstream, the contrast agent helps to highlight specific areas and improve the differentiation between organs, lesions, and abnormalities.
In a recent case study, a CTA was conducted to aid in surgical planning for a Golden Retriever diagnosed with an expansile multilobulated granular bony calvarium mass. The CTA facilitated vascular mapping and precise localisation of the tumour, enabling the neurosurgeon to assess the feasibility of undertaking surgery of this magnitude and evaluate potential associated complications.
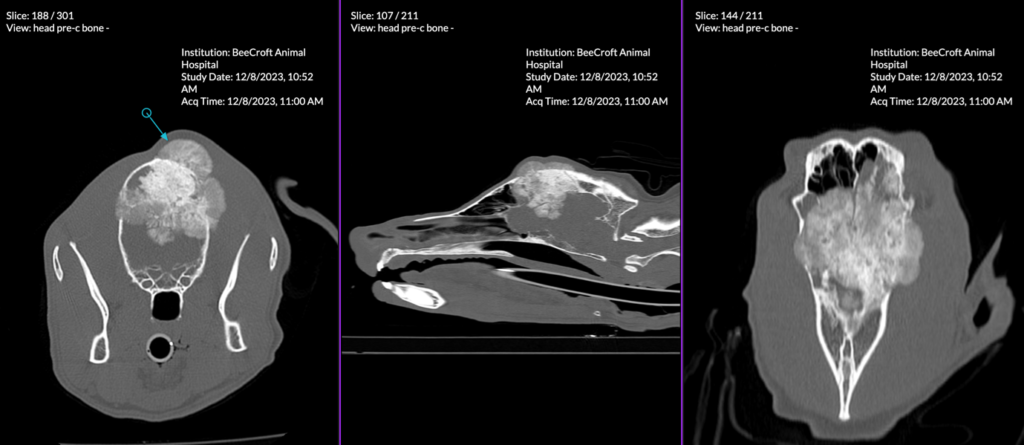
Figure 3. Multiplanar reconstruction of a multilobulated expansile mineralised mass-like lesion at the calvarium that has significantly impacted adjacent structures.
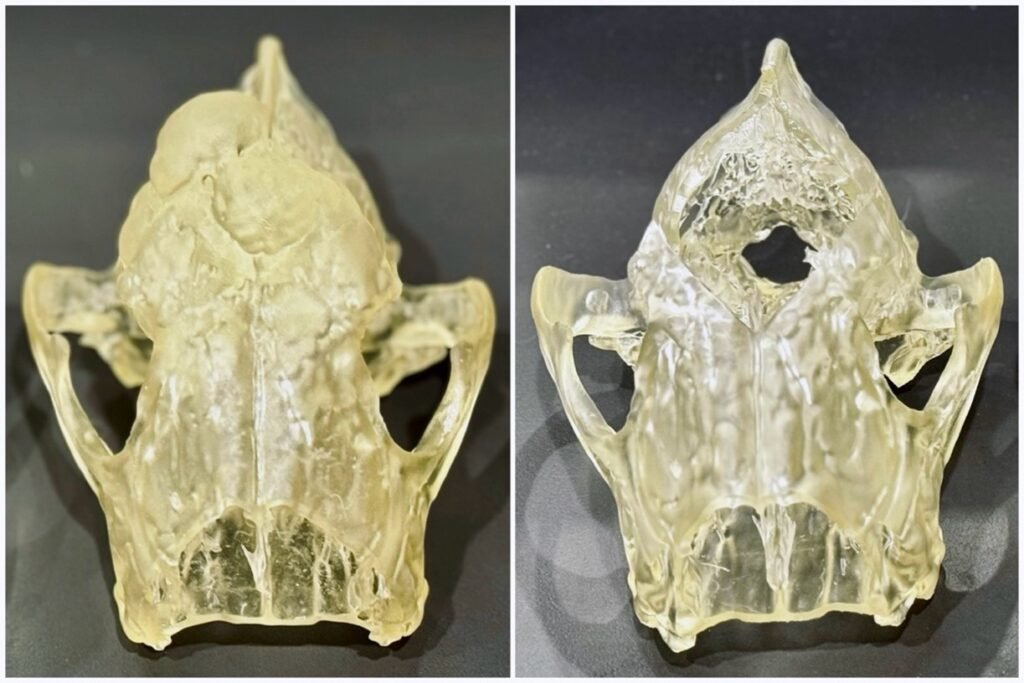
Figure 4. 3D-printed model of cranium with a multilobulated expansile mineralised mass-like lesion (left) and 3D-printed model of cranium with simulated surgical tumour removal (right).
Shunts and Vascular Anomalies
Multiphase abdominal contrast studies, comprising arterial, portal, venous, and delayed phases, serve to characterise lesions or anomalies based on contrast enhancement at distinct time intervals. Various methods are employed for acquiring contrast-enhanced CTA, each presenting its advantages and disadvantages. Notably, injection protocols utilising test bolus tracking necessitate a larger contrast medium volume and extended anaesthesia time to attain precise peak enhancement. This approach facilitates increased accuracy in results, considering the potential impact of patient variability on the corresponding timings for vascular phases. Despite being more labour and time-intensive, test bolus tracking can overcome the variations in contrast enhancement patterns affected by cardiac output and cardiovascular circulation that differ in every patient.
In comparison, bolus tracking represents a time-efficient approach whereby the contrast bolus’s transit through the vasculature is monitored to ascertain the ideal moment for image acquisition. A designated region of interest (ROI) is positioned within a major artery or vein, with the CT scanner continually assessing the CT attenuation (density) within this region. Upon reaching a predetermined threshold of contrast concentration in the targeted vessels, the scan commences automatically, thus reducing the necessity for manual intervention and ensuring consistency in timing. Nonetheless, depending on the desired phase acquisition, certain scenarios may entail a delay between the arrival of the contrast bolus and the initiation of the scan, potentially resulting in suboptimal timing and compromising image quality.
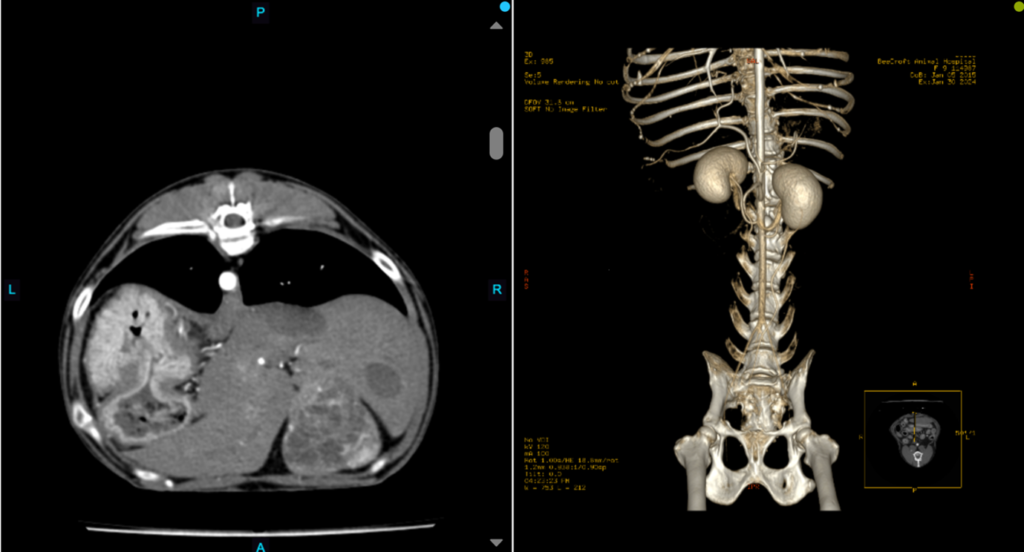
Figure 5. A transverse view of a canine abdomen during the arterial phase. Notable hyperintensity was observed exclusively within the aorta, with no corresponding hyperintensity evident in the caudal vena cava (left). Volume rending of the same arterial phase with visible hyperintense aorta and other arteries (right).
Nasal and Oral Lesions
CT offers exceptionally detailed imaging of nasal structures, facilitating a thorough examination of the nasal cavity, paranasal sinuses, and adjacent tissues. This heightened level of detail is instrumental in the identification and characterisation of diverse nasal pathologies, encompassing tumours, inflammatory processes, and developmental anomalies. Moreover, CT serves as a valuable tool for evaluating the extent of bony involvement in nasal lesions, furnishing intricate insights into bone destruction, erosion patterns, and remodelling changes. Such detailed information is indispensable for accurately gauging disease extent and strategising interventions, including rhinoscopies and surgical procedures.
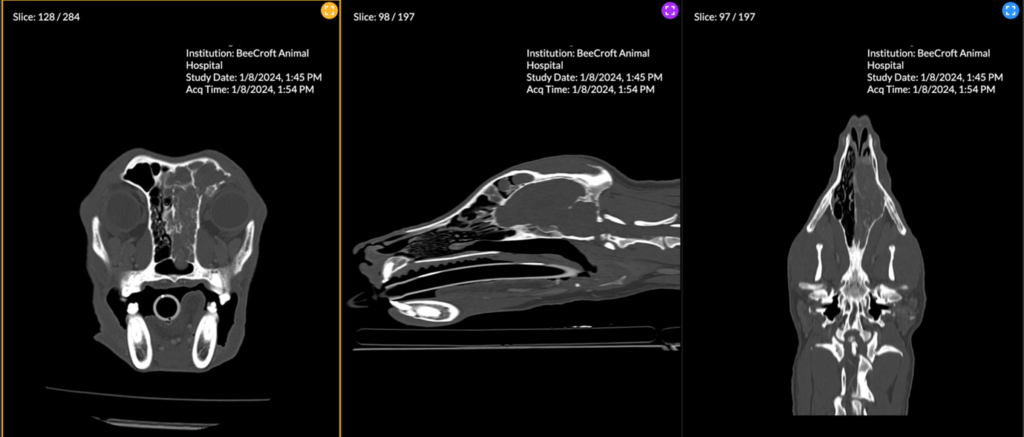
Figure 6. A multiplanar view of a large heterogenous soft tissue attenuating mass occupying a majority of the right nasal cavity, right frontal sinus and sphenoidal sinus. Extensive lysis of the nasal turbinates, medial wall of the right orbit, right frontal bone, ethmoidal bone, nasal septum, and cribriform plate has resulted from aggressive neoplastic invasion.
CONE BEAM COMPUTED TOMOGRAPHY CANNOT SUBSTITUTE CT
It is important not to confuse Cone Beam Computed Tomography (CBCT) with conventional CT imaging. CBCT utilises a cone-shaped X-ray beam and a two-dimensional detector to capture images in a single rotation around the patient. Its primary application is dental and facial imaging, providing views of teeth and bones in the facial area. However, there are limitations to its utility. CBCT is not recommended for routine imaging of thoracic and abdominal regions or soft tissue examinations due to its reduced soft tissue contrast resolution and increased motion artefacts. Cone beam technology is not reliable for tissue density assessment, reducing the ability to define soft tissue lesions or structures (Varshowsaz, et al., 2016).
On the other hand, conventional CT uses multiple detectors to capture images from various angles and perform multiple rotations around the animal. Conventional CT is particularly suited for comprehensive imaging of the skull, brain, and larger anatomical regions like the thorax, abdomen, or spine. As a result, CT offers greater diagnostic capabilities compared to CBCT and cannot be replaced by it.
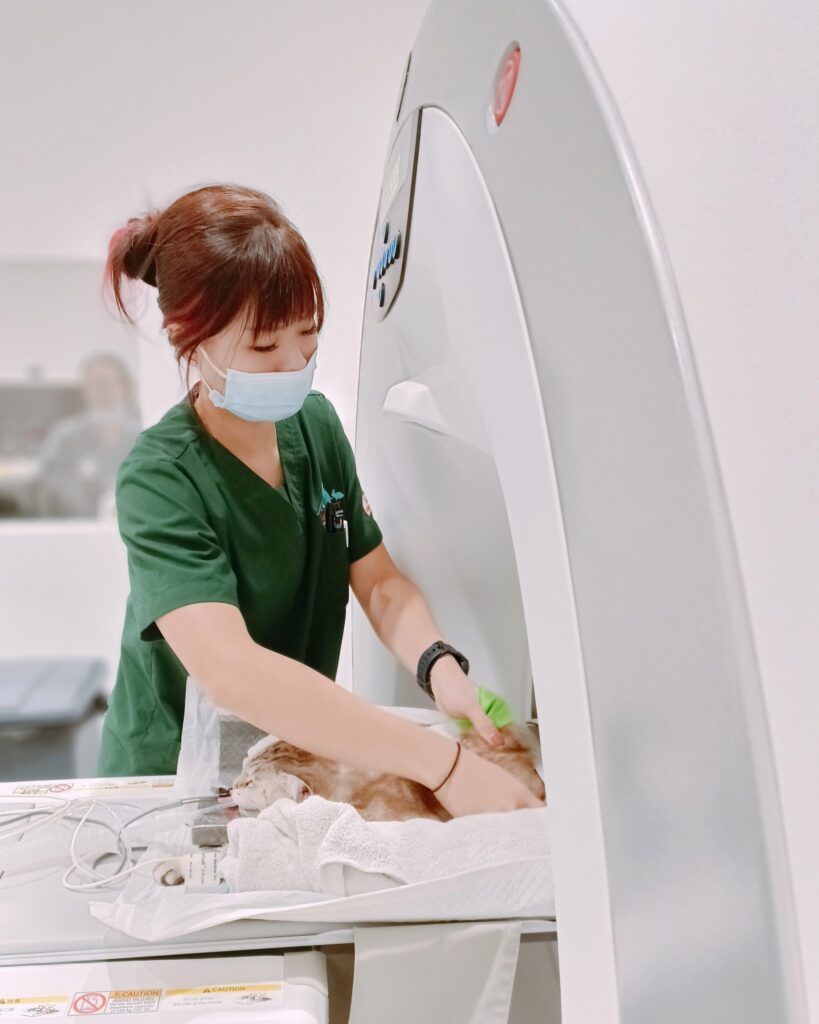
Figure 7. Arabel Yap, head of Imaging department at Beecroft, positions a patient on the CT table to ensure the area of scan is properly aligned with the scanner’s gantry.
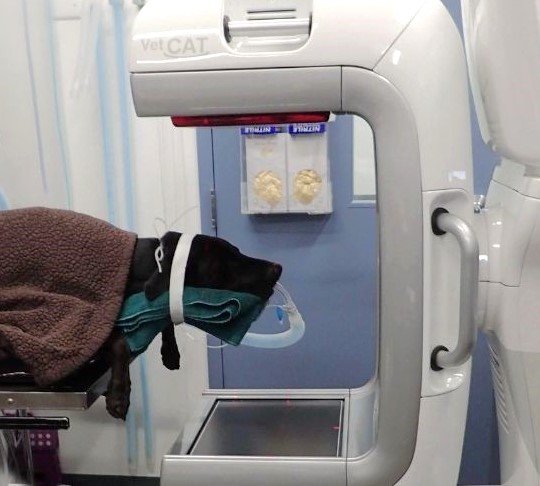
References
- Brühschwein, A., Schmitz, B., Zöllner, M., Reese, S., & Meyer-Lindenberg, A. (2023). Three-dimensional computed tomographic angular measurements of the canine tibia using a bone-centered coordinate system. Frontiers in Veterinary Science, 10. https://doi.org/10.3389/fvets.2023.1154144
- Choi, S., Lee, I., Choi, H., Lee, K., Park, I., & Lee, Y. (2018). Establishment of Injection Protocol of test bolus for precise scan timing in canine abdominal multi-phase computed tomography. Journal of Veterinary Clinics, 35(3), 93–96. https://doi.org/10.17555/jvc.2018.06.35.3.93
- Davé, A. C., Ober, C. P., & Rendahl, A. (2022, July 1). Factors influencing enhancement timing in a triple-phase abdominal CT angiography protocol in dogs. AVMA. https://avmajournals.avma.org/view/journals/ajvr/83/7/ajvr.21.03.0031.xml
- Greco, A., Meomartino, L., Gnudi, G., Brunetti, A., & Di Giancamillo, M. (2022). Imaging techniques in veterinary medicine. part II: Computed Tomography, Magnetic Resonance Imaging, Nuclear Medicine. European Journal of Radiology Open, 10, 100467. https://doi.org/10.1016/j.ejro.2022.100467
- Hebel, M., Panek, W. K., Ruszkowski, J. J., Nabzdyk, M., Niedzielski, D., Pituch, K. C., Jackson, A. M., Kiełbowicz, M., & Pomorska-Mól, M. (2021). Computed tomography findings in a cohort of 169 dogs with elbow dysplasia – a retrospective study. BMC Veterinary Research, 17(1). https://doi.org/10.1186/s12917-021-02997-5
- Lau, S. F., Wolschrijn, C. F., Hazewinkel, H. A. W., Siebelt, M., & Voorhout, G. (2013). The early development of medial coronoid disease in growing Labrador retrievers: Radiographic, computed tomographic, necropsy and micro-computed tomographic findings. The Veterinary Journal, 197(3), 724–730. https://doi.org/10.1016/j.tvjl.2013.04.002
- Meomartino, L., Greco, A., Di Giancamillo, M., Brunetti, A., & Gnudi, G. (2021). Imaging techniques in Veterinary Medicine. Part I: Radiography and Ultrasonography. European journal of radiology open, 8, 100382. https://doi.org/10.1016/j.ejro.2021.100382
- Varshowsaz, M., Rahimian, S., Goorang, S., Azizi, Z., & Ehsani, S. (2016, March). Comparison of tissue density in Hounsfield units in computed tomography and cone beam computed tomography. Journal of dentistry (Tehran, Iran). https://pubmed.ncbi.nlm.nih.gov/27928239/